Home>Education & Research>PCR Steps: A Comprehensive Guide To Polymerase Chain Reaction
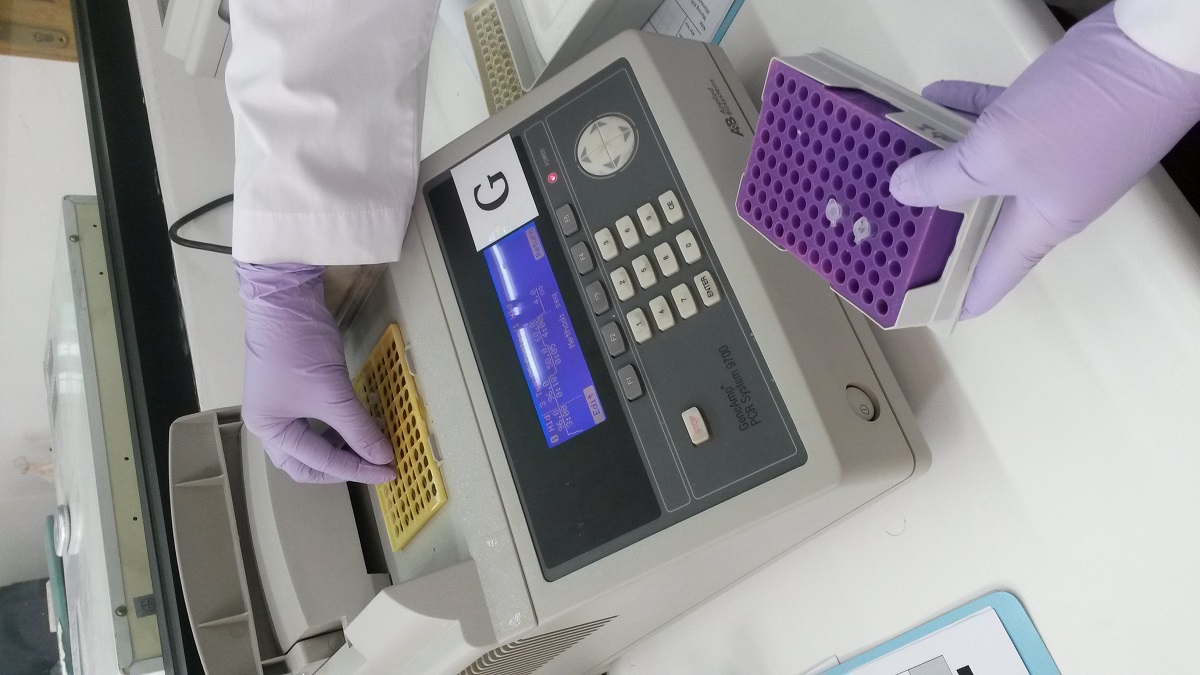
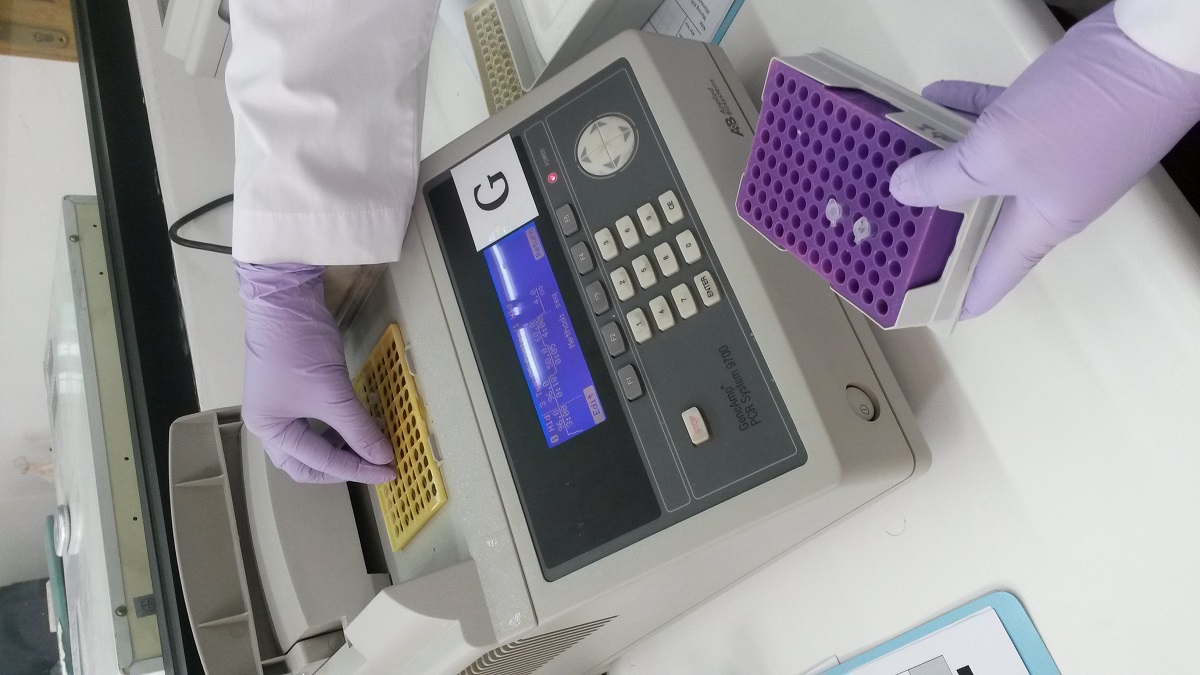
Education & Research
PCR Steps: A Comprehensive Guide To Polymerase Chain Reaction
Published: February 19, 2024
Learn the essential PCR steps for Education & Research with this comprehensive guide. Master the techniques and principles of Polymerase Chain Reaction.
(Many of the links in this article redirect to a specific reviewed product. Your purchase of these products through affiliate links helps to generate commission for Temperatures.com, at no extra cost. Learn more)
Introduction
Polymerase chain reaction (PCR) is a revolutionary technique that has transformed the field of molecular biology and genetics. This powerful tool allows researchers to amplify a specific segment of DNA, generating millions of copies from just a single or a few DNA molecules. The ability to produce a large quantity of DNA from a minute sample has numerous applications in various scientific disciplines, including medical diagnostics, forensic analysis, evolutionary biology, and genetic engineering.
PCR is a cornerstone of modern molecular biology research, enabling scientists to study and manipulate DNA with unprecedented precision and efficiency. The technique was first developed in the 1980s by Kary Mullis, who was awarded the Nobel Prize in Chemistry in 1993 for his groundbreaking contribution to the field. Since its inception, PCR has become an indispensable tool in laboratories worldwide, driving advancements in diverse areas of biological research.
The fundamental principle of PCR lies in its ability to exponentially amplify a specific DNA sequence through a series of temperature-controlled cycles. This process involves three key steps: denaturation, annealing, and extension, which are meticulously orchestrated to achieve targeted DNA replication. Each step is facilitated by a specialized DNA polymerase enzyme and requires precise temperature control to ensure the success of the amplification process.
The widespread adoption of PCR has revolutionized genetic analysis, enabling the detection of genetic diseases, identification of pathogens, and analysis of ancient DNA samples. Moreover, PCR has played a pivotal role in the development of various biotechnological applications, such as the production of recombinant DNA, gene cloning, and the synthesis of specific DNA fragments for research and therapeutic purposes.
In this comprehensive guide, we will delve into the intricacies of each PCR step, unraveling the molecular choreography that underpins this transformative technique. By understanding the principles and nuances of PCR, researchers and enthusiasts alike can gain a deeper appreciation for the elegance and power of this molecular tool. Join us on this journey as we unravel the inner workings of PCR and explore its profound impact on the realm of molecular biology and beyond.
Step 1: Denaturation
During the first phase of the polymerase chain reaction (PCR) process, known as denaturation, the double-stranded DNA template is exposed to high temperatures, typically around 94-98°C. This elevated temperature causes the hydrogen bonds between the complementary nucleotide base pairs (adenine-thymine and guanine-cytosine) to break, leading to the separation of the two DNA strands. As a result, the double-stranded DNA molecule unravels into two single-stranded templates, setting the stage for the subsequent steps of the PCR cycle.
The denaturation step is crucial as it ensures that the DNA strands are fully separated, allowing the primers to bind to their complementary sequences during the annealing phase. This process is facilitated by the use of a specialized DNA polymerase enzyme, such as Taq polymerase, which exhibits remarkable heat resistance and remains active at the high temperatures required for denaturation.
The denaturation phase is typically maintained for a short duration, usually lasting between 20 to 30 seconds, to prevent the DNA from becoming irreversibly damaged due to prolonged exposure to high temperatures. The precise timing and temperature control during denaturation are essential to ensure the efficient separation of the DNA strands while preserving the integrity of the genetic material.
From a molecular perspective, the denaturation step mimics the natural process of DNA unwinding that occurs during DNA replication and transcription in living organisms. By harnessing this fundamental biological phenomenon, PCR enables researchers to initiate the amplification of specific DNA sequences with unparalleled precision and reproducibility.
The denaturation step serves as the initial act in the PCR symphony, setting the stage for the subsequent steps of annealing and extension. Through this orchestrated process, PCR empowers scientists to exponentially replicate targeted DNA sequences, unlocking a myriad of applications across diverse scientific disciplines.
In the next section, we will explore the intricacies of the annealing step, shedding light on the pivotal role of primers in guiding the amplification of specific DNA regions during the PCR cycle. Join us as we unravel the molecular intricacies that drive the remarkable capabilities of polymerase chain reaction.
Step 2: Annealing
Following the denaturation phase, the polymerase chain reaction (PCR) enters the critical stage of annealing, where the temperature is lowered to facilitate the binding of short DNA primers to the complementary sequences on the single-stranded DNA templates. The primers, typically 18-22 nucleotides in length, are designed to specifically anneal to the regions flanking the target DNA sequence, serving as the starting point for DNA synthesis by the DNA polymerase enzyme.
The annealing temperature is carefully optimized to ensure the specificity and efficiency of primer binding to the target DNA. This temperature typically ranges between 50-65°C, depending on the length and nucleotide composition of the primers and the target DNA sequence. During annealing, the hydrogen bonds form between the complementary nucleotide base pairs, allowing the primers to anneal to their specific binding sites on the single-stranded DNA templates.
The precise annealing of the primers is a pivotal step in the PCR process, as it dictates the specificity and selectivity of DNA amplification. By designing primers with sequences that are complementary to the target DNA, researchers can ensure that the amplification is directed towards the desired genetic region, minimizing non-specific amplification and enhancing the accuracy of the PCR results.
Moreover, the annealing phase plays a crucial role in determining the efficiency of the subsequent DNA synthesis during the extension step. Proper primer annealing sets the stage for the DNA polymerase enzyme to initiate the synthesis of a new DNA strand, using the annealed primers as the starting point for DNA replication.
The orchestration of the annealing step is a testament to the precision and intricacy of PCR, where the interplay of temperature, primer design, and DNA template specificity converges to drive the targeted amplification of DNA sequences. Through this delicate molecular dance, PCR empowers researchers to selectively amplify and study specific regions of the genome, unlocking a wealth of possibilities in genetic analysis, diagnostics, and research.
As the annealing phase sets the foundation for the subsequent extension step, it serves as a pivotal juncture in the PCR cycle, shaping the trajectory of DNA amplification and laying the groundwork for the exponential replication of the target DNA sequence. Join us as we delve deeper into the final step of the PCR cycle, where the synthesis of new DNA strands unfolds with remarkable precision and efficiency.
Step 3: Extension
In the culmination of the polymerase chain reaction (PCR) cycle, the pivotal phase of extension unfolds, marking the initiation of DNA synthesis by the DNA polymerase enzyme. This transformative step is characterized by the amplification of the target DNA sequence through the synthesis of a complementary DNA strand, driven by the precise coordination of temperature and enzymatic activity.
As the temperature is raised to the optimal range of 72-78°C, the DNA polymerase enzyme, often the renowned Taq polymerase derived from the thermophilic bacterium Thermus aquaticus, catalyzes the addition of nucleotides to the annealed primers, extending the DNA strand in a template-dependent manner. The enzyme's remarkable heat stability allows it to withstand the elevated temperatures required for DNA synthesis, making it an indispensable component of the PCR process.
During the extension phase, the DNA polymerase enzyme incorporates nucleotides, building a complementary DNA strand along each of the single-stranded DNA templates. This process follows the principles of complementary base pairing, where adenine (A) pairs with thymine (T) and cytosine (C) pairs with guanine (G), ensuring the accurate replication of the target DNA sequence. The DNA polymerase's fidelity in incorporating the correct nucleotides contributes to the precision and reliability of DNA amplification in PCR.
The duration of the extension phase is influenced by the length of the DNA target and the DNA polymerase's elongation rate, typically ranging from 30 seconds to several minutes. The optimal extension time is carefully determined to ensure the completion of DNA synthesis across all target sequences, balancing efficiency with the fidelity of DNA replication.
As the extension phase draws to a close, the DNA polymerase faithfully synthesizes new DNA strands, mirroring the natural process of DNA replication in living organisms. The coordinated interplay of temperature, enzymatic activity, and nucleotide incorporation culminates in the exponential amplification of the target DNA, yielding millions of copies from the initial DNA template.
The precision and efficiency of the extension phase underscore the remarkable capabilities of PCR, enabling researchers to selectively amplify and analyze specific DNA sequences with unparalleled accuracy and reproducibility. Through this orchestrated molecular symphony, PCR has revolutionized genetic analysis, diagnostics, and research, empowering scientists to unravel the intricacies of the genome and harness the potential of DNA in diverse scientific endeavors.
As we conclude our exploration of the extension phase, we invite you to reflect on the profound impact of PCR in driving advancements in molecular biology and genetics. Join us in celebrating the elegance and power of this transformative technique, as we continue to unravel the mysteries of the genetic code and its myriad applications in the realm of science and beyond.
Conclusion
In conclusion, the polymerase chain reaction (PCR) stands as a cornerstone of modern molecular biology, revolutionizing genetic analysis, diagnostics, and research endeavors. The intricate orchestration of denaturation, annealing, and extension, under precise temperature control, empowers researchers to exponentially amplify specific DNA sequences with unparalleled precision and efficiency.
Through the denaturation phase, the double-stranded DNA unravels into single-stranded templates, setting the stage for the subsequent steps of the PCR cycle. This critical step mimics the natural process of DNA unwinding, laying the foundation for targeted DNA replication.
The annealing phase, characterized by the precise binding of primers to the complementary DNA sequences, dictates the specificity and selectivity of DNA amplification. This pivotal step ensures that the amplification is directed towards the desired genetic region, minimizing non-specific amplification and enhancing the accuracy of PCR results.
The culmination of the PCR cycle unfolds in the extension phase, where DNA synthesis is initiated by the DNA polymerase enzyme. The enzyme's fidelity in incorporating the correct nucleotides contributes to the precision and reliability of DNA replication, culminating in the exponential amplification of the target DNA.
The transformative impact of PCR extends across diverse scientific disciplines, enabling the detection of genetic diseases, identification of pathogens, and analysis of ancient DNA samples. Moreover, PCR has played a pivotal role in the development of various biotechnological applications, such as gene cloning, recombinant DNA production, and the synthesis of specific DNA fragments for research and therapeutic purposes.
As we reflect on the profound impact of PCR, it becomes evident that this transformative technique has unlocked a wealth of possibilities in genetic analysis and molecular biology. The precision, reproducibility, and versatility of PCR have propelled advancements in genetics, medicine, forensics, and evolutionary biology, shaping our understanding of the genetic code and its implications in diverse scientific endeavors.
In essence, the elegance and power of PCR lie in its ability to unravel the mysteries of the genome, enabling researchers to decode the genetic blueprint with unprecedented accuracy and efficiency. As we celebrate the profound impact of PCR, we are poised to witness further innovations and discoveries, driven by the remarkable capabilities of this transformative molecular tool.