Home>Science & Environment>The Transfer Of Thermal Energy From Frozen Ice To Another Substance: Explained
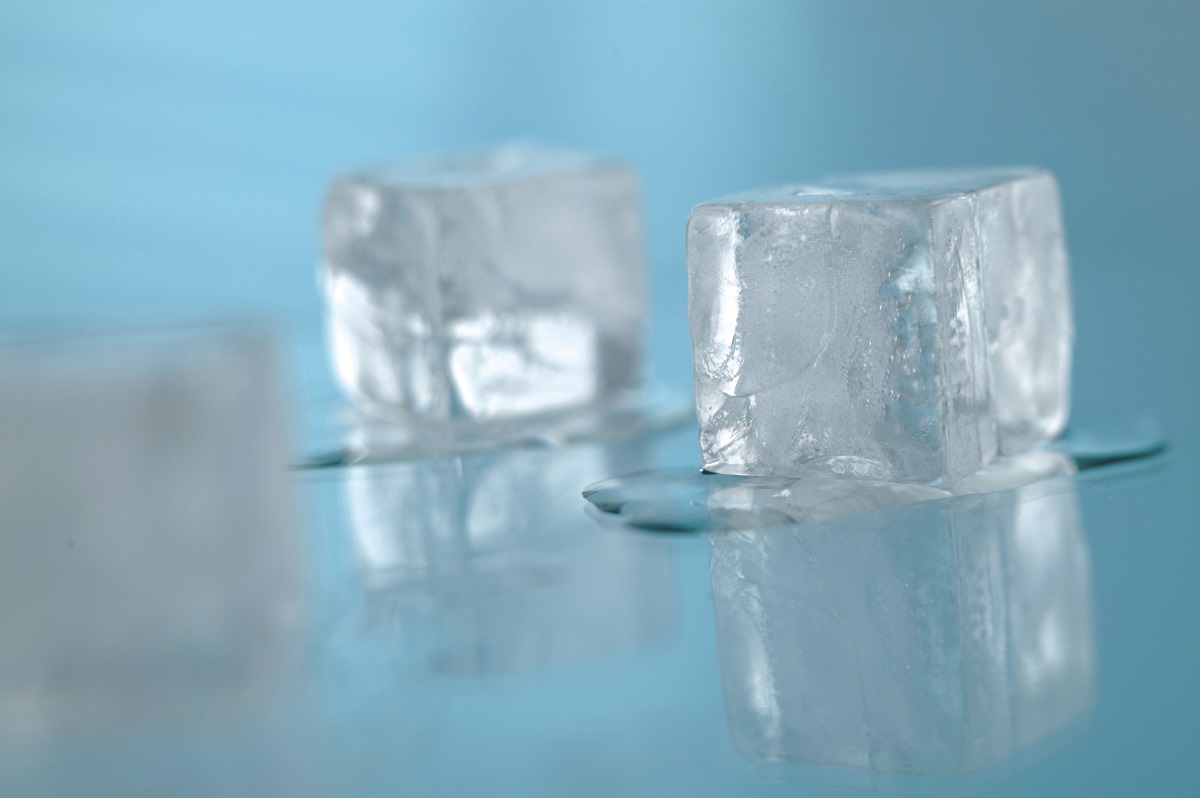
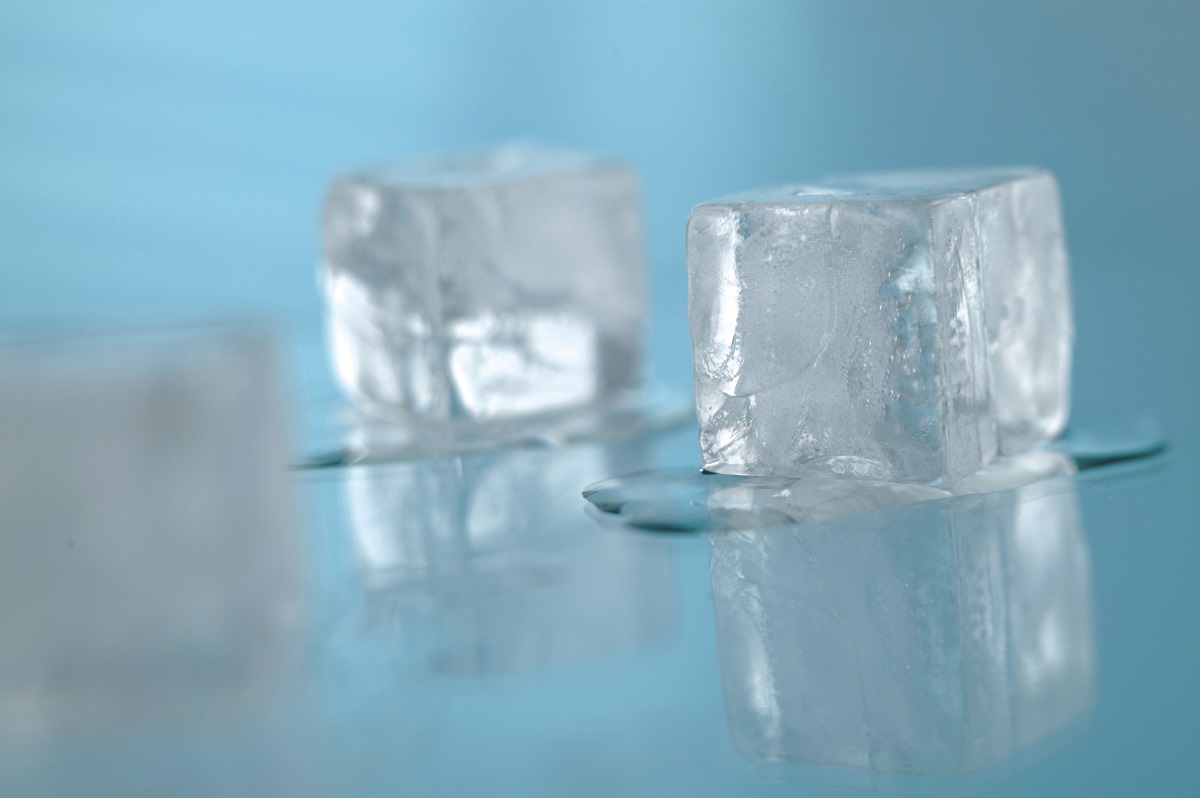
Science & Environment
The Transfer Of Thermal Energy From Frozen Ice To Another Substance: Explained
Published: February 19, 2024
Learn about the transfer of thermal energy from frozen ice to other substances in this comprehensive guide. Explore the science and environmental impact of this process.
(Many of the links in this article redirect to a specific reviewed product. Your purchase of these products through affiliate links helps to generate commission for Temperatures.com, at no extra cost. Learn more)
Table of Contents
Introduction
The transfer of thermal energy from frozen ice to another substance is a fascinating process that occurs in various natural and human-made scenarios. Thermal energy transfer, also known as heat transfer, is a fundamental concept in the field of science and plays a crucial role in our daily lives. Understanding how thermal energy is transferred from frozen ice to other substances is not only intriguing but also essential for comprehending numerous phenomena and applications.
In this article, we will delve into the intricate mechanisms of thermal energy transfer from frozen ice, exploring the factors that influence this process and its diverse applications. By unraveling the science behind this phenomenon, we can gain a deeper appreciation for the fundamental principles governing thermal energy transfer and its significance in different contexts.
The transfer of thermal energy from frozen ice to another substance is a dynamic process that involves the exchange of heat between the ice and the surrounding environment. This exchange of thermal energy occurs through various mechanisms, each with its unique characteristics and implications. By examining these mechanisms, we can gain insights into the underlying principles that govern thermal energy transfer and its impact on the behavior of different substances.
As we embark on this exploration, we will uncover the intricate interplay of factors that influence thermal energy transfer from frozen ice, shedding light on the complex dynamics at play. From the molecular level interactions to macroscopic phenomena, the transfer of thermal energy from frozen ice encompasses a rich tapestry of scientific principles and practical implications.
Join us on this enlightening journey as we unravel the mysteries of thermal energy transfer from frozen ice and discover its profound significance in the world of science and beyond. Through this exploration, we aim to foster a deeper understanding of this captivating process and its far-reaching implications in diverse fields, from environmental science to engineering and beyond.
Understanding Thermal Energy Transfer
Thermal energy transfer, also known as heat transfer, is a fundamental process that underpins numerous natural phenomena and technological applications. At its core, thermal energy transfer involves the movement of heat from a region of higher temperature to a region of lower temperature. This exchange of thermal energy occurs through various mechanisms, each with its distinct characteristics and implications.
The three primary modes of thermal energy transfer are conduction, convection, and radiation. Conduction involves the transfer of heat through direct molecular collisions within a material or between different materials in contact. This mode of heat transfer is prevalent in solids and occurs as the vibrating molecules pass on their kinetic energy to neighboring particles, propagating the thermal energy through the material.
Convection, on the other hand, involves the transfer of heat through the movement of fluids, such as liquids and gases. This mode of heat transfer is driven by the circulation of the fluid, which carries thermal energy from one location to another. Convection plays a significant role in atmospheric processes, ocean currents, and various industrial applications where fluid dynamics influence heat transfer.
Radiation, the third mode of thermal energy transfer, occurs through electromagnetic waves that propagate through space. Unlike conduction and convection, radiation does not require a medium for heat transfer and can occur in a vacuum. This mode of heat transfer is responsible for the transmission of solar energy, the emission of thermal radiation from objects, and the propagation of electromagnetic waves in various forms.
Understanding the mechanisms of thermal energy transfer is crucial for comprehending the behavior of different substances and the dynamics of heat exchange in various environments. Whether it's the conduction of heat through a metal rod, the convection-driven circulation of air in a room, or the radiation of heat from the sun, thermal energy transfer shapes our physical world and influences a myriad of natural and engineered systems.
By unraveling the intricacies of thermal energy transfer, scientists and engineers can develop innovative solutions for managing heat in diverse applications, from thermal insulation and energy-efficient technologies to climate modeling and thermal management in electronic devices. The profound impact of thermal energy transfer extends across disciplines, making it a cornerstone of scientific inquiry and technological advancement.
In the next section, we will explore the specific process of thermal energy transfer from frozen ice, delving into the unique dynamics and implications of this fascinating phenomenon. Through this exploration, we will gain deeper insights into the interplay of factors that govern the transfer of thermal energy from frozen ice to other substances, shedding light on its significance in various contexts.
The Process of Thermal Energy Transfer from Frozen Ice
The process of thermal energy transfer from frozen ice involves intricate mechanisms that govern the exchange of heat between the ice and its surroundings. When frozen ice comes into contact with a substance at a higher temperature, such as water or air, thermal energy transfer occurs through the fundamental principles of heat exchange. This process is driven by the temperature difference between the ice and the surrounding medium, leading to the flow of heat from the warmer substance to the colder ice.
One of the primary modes of thermal energy transfer from frozen ice is conduction. As the warmer substance, whether it's water or air, comes into contact with the surface of the ice, heat is transferred through direct molecular collisions. The kinetic energy of the molecules in the warmer substance is imparted to the molecules at the interface of the ice, causing them to vibrate and propagate the thermal energy through the ice. This conduction process continues until thermal equilibrium is reached, with the ice absorbing heat and undergoing a phase transition from solid to liquid.
Additionally, the process of thermal energy transfer from frozen ice can also involve convection, especially in the case of ice melting in a liquid medium. As the ice absorbs heat from the surrounding liquid, the warmer liquid near the ice surface rises due to decreased density, creating a convection current that facilitates the transfer of thermal energy. This convective heat transfer accelerates the melting of the ice and contributes to the redistribution of thermal energy within the liquid medium.
Furthermore, radiation plays a role in the thermal energy transfer from frozen ice, particularly in scenarios where the ice is exposed to radiant heat sources, such as sunlight. In such cases, the ice absorbs thermal radiation, leading to an increase in its temperature and the subsequent transfer of heat to the surrounding environment.
The interplay of conduction, convection, and radiation in the process of thermal energy transfer from frozen ice underscores the dynamic nature of heat exchange and its implications for the behavior of ice and the surrounding substances. By unraveling the intricacies of this process, scientists and engineers can develop insights into managing thermal energy in diverse contexts, from cryogenic applications to environmental processes influenced by ice dynamics.
Understanding the process of thermal energy transfer from frozen ice is essential for addressing challenges related to climate change, cryopreservation, and thermal management in various industries. By gaining a deeper appreciation for the mechanisms governing this process, we can harness its principles to develop innovative solutions that leverage the unique properties of frozen ice and its role in heat exchange phenomena.
Factors Affecting Thermal Energy Transfer from Frozen Ice
Several factors influence the thermal energy transfer process from frozen ice to other substances, shaping the dynamics of heat exchange and the behavior of the ice-solid phase. Understanding these factors is crucial for comprehending the complexities of thermal energy transfer and its implications in diverse scenarios.
-
Temperature Differential: The temperature gradient between the frozen ice and the surrounding medium significantly impacts the rate of thermal energy transfer. A larger temperature differential accelerates the heat exchange, leading to faster melting of the ice and a more pronounced transfer of thermal energy.
-
Thermal Conductivity: The thermal conductivity of the materials involved, including the frozen ice and the contacting substance, plays a pivotal role in determining the efficiency of heat transfer. Materials with higher thermal conductivity facilitate more rapid heat exchange, influencing the overall rate of thermal energy transfer from the ice.
-
Surface Area and Contact Geometry: The surface area of contact between the frozen ice and the surrounding substance, along with the specific contact geometry, influences the extent of heat transfer. A larger contact area allows for greater heat exchange, while the nature of the contact interface can impact the efficiency of thermal energy transfer.
-
Physical State of the Surrounding Medium: The physical state of the surrounding medium, whether it's a liquid or a gas, can affect the mechanism of heat transfer from the frozen ice. Convection-driven heat transfer is more pronounced in liquids, while conduction and radiation play distinct roles in gases, influencing the overall dynamics of thermal energy transfer.
-
Presence of Insulating Materials: The presence of insulating materials in the vicinity of the frozen ice can impede heat transfer by reducing the thermal conductivity and altering the temperature gradient. Insulation can significantly impact the rate of thermal energy transfer, leading to slower melting of the ice and a modified heat exchange process.
-
Environmental Conditions: Environmental factors, such as ambient temperature, humidity, and the presence of radiant heat sources, can influence the thermal energy transfer from frozen ice. These conditions can modulate the rate and direction of heat flow, shaping the overall dynamics of heat exchange in the surrounding environment.
By considering these factors, scientists and engineers can gain valuable insights into optimizing thermal energy transfer processes involving frozen ice. Whether it's designing efficient cooling systems, understanding the behavior of ice in natural environments, or developing innovative cryogenic technologies, the interplay of these factors shapes the intricate dynamics of thermal energy transfer from frozen ice.
Applications of Thermal Energy Transfer from Frozen Ice
The process of thermal energy transfer from frozen ice holds significant relevance in various practical applications, leveraging the unique properties of ice and its role in heat exchange phenomena. Understanding these applications provides valuable insights into harnessing the principles of thermal energy transfer for diverse purposes, ranging from environmental processes to technological innovations.
Cryopreservation and Biomedical Research
In the field of cryopreservation, thermal energy transfer from frozen ice plays a crucial role in preserving biological samples, tissues, and cells at ultra-low temperatures. By carefully managing the heat exchange between the frozen ice and the biological specimens, scientists can achieve controlled cooling rates and minimize cellular damage during the freezing process. This application is instrumental in biomedical research, organ transplantation, and the long-term storage of biological materials, offering a pathway for advancing regenerative medicine and biotechnology.
Cold Chain Logistics and Food Preservation
The thermal energy transfer from frozen ice finds extensive use in cold chain logistics and food preservation, where maintaining low temperatures is essential for preserving the quality and safety of perishable goods. From frozen food storage and transportation to the distribution of temperature-sensitive pharmaceuticals, the controlled transfer of thermal energy from frozen ice enables the preservation of products, ensuring their integrity and extending their shelf life. This application is critical for ensuring food security, reducing food waste, and safeguarding the efficacy of medical supplies.
Climate Modeling and Glaciology
In the realm of climate modeling and glaciology, understanding the dynamics of thermal energy transfer from frozen ice is essential for simulating ice melt processes, predicting sea level rise, and assessing the impact of climate change on polar regions. By incorporating accurate representations of heat exchange between ice masses and their surroundings, scientists can refine climate models and improve projections of ice sheet behavior, contributing to our understanding of global climate dynamics and the implications for sea level variability.
Cryogenic Engineering and Superconductivity
In the field of cryogenic engineering, the controlled transfer of thermal energy from frozen ice is instrumental in creating and maintaining ultra-low temperatures for various applications, including superconducting technologies. By leveraging the principles of heat exchange, engineers can design efficient cryogenic systems for cooling superconducting magnets, particle accelerators, and quantum computing devices, unlocking the transformative potential of superconductivity in advancing energy infrastructure, scientific research, and information technology.
Read more: Different Types Of Heat Transfer
Environmental Monitoring and Ice Dynamics
In environmental monitoring and ice dynamics research, thermal energy transfer from frozen ice serves as a fundamental mechanism for understanding the behavior of ice formations, such as glaciers, ice shelves, and sea ice. By studying the heat exchange processes at the ice-atmosphere and ice-ocean interfaces, scientists can gain insights into the thermal evolution of ice masses, contributing to assessments of polar ice melt, freshwater discharge, and the interactions between ice and the surrounding environment. This application is pivotal for elucidating the impacts of climate change on polar regions and global hydrological cycles.
Innovative Cooling Technologies and Energy Efficiency
The principles of thermal energy transfer from frozen ice inspire the development of innovative cooling technologies and energy-efficient systems. From phase change materials that utilize ice as a thermal reservoir to advanced refrigeration and air conditioning designs, the controlled manipulation of heat exchange processes involving frozen ice drives advancements in sustainable cooling solutions, contributing to reduced energy consumption, lower carbon emissions, and enhanced thermal comfort in diverse settings.
By exploring these applications, we gain a deeper appreciation for the multifaceted role of thermal energy transfer from frozen ice in shaping diverse fields, from biomedicine and environmental science to engineering and climate research. The intricate interplay of heat exchange dynamics involving frozen ice underscores its profound significance in addressing global challenges and driving technological innovation.
Conclusion
The transfer of thermal energy from frozen ice to other substances encompasses a rich tapestry of scientific principles, practical applications, and environmental implications. Through our exploration of this captivating process, we have unveiled the intricate mechanisms that govern heat exchange involving frozen ice, shedding light on the dynamic interplay of conduction, convection, and radiation in the transfer of thermal energy. From cryopreservation and cold chain logistics to climate modeling and cryogenic engineering, the applications of thermal energy transfer from frozen ice span diverse domains, showcasing its profound relevance in addressing global challenges and driving technological innovation.
By delving into the factors that influence thermal energy transfer from frozen ice, we have gained valuable insights into optimizing heat exchange processes and harnessing the unique properties of ice for various applications. The temperature gradient, thermal conductivity, contact geometry, and environmental conditions all play pivotal roles in shaping the dynamics of heat exchange, offering avenues for enhancing the efficiency and sustainability of thermal energy transfer involving frozen ice.
Furthermore, our exploration of the applications of thermal energy transfer from frozen ice has underscored its pivotal role in biomedical research, food preservation, climate modeling, cryogenic engineering, environmental monitoring, and sustainable cooling technologies. The controlled management of heat exchange processes involving frozen ice has far-reaching implications for advancing healthcare, ensuring food security, understanding climate dynamics, enabling cutting-edge technologies, and fostering environmental stewardship.
In conclusion, the transfer of thermal energy from frozen ice represents a captivating intersection of scientific inquiry, technological innovation, and environmental significance. By unraveling the complexities of this process and embracing its diverse applications, we can harness the principles of heat exchange to address pressing global challenges, drive sustainable development, and unlock the transformative potential of thermal energy transfer from frozen ice in shaping a resilient and interconnected world.