Home>Science & Environment>Exploring The Relationship Between Temperature And Resistance In Conducting Materials
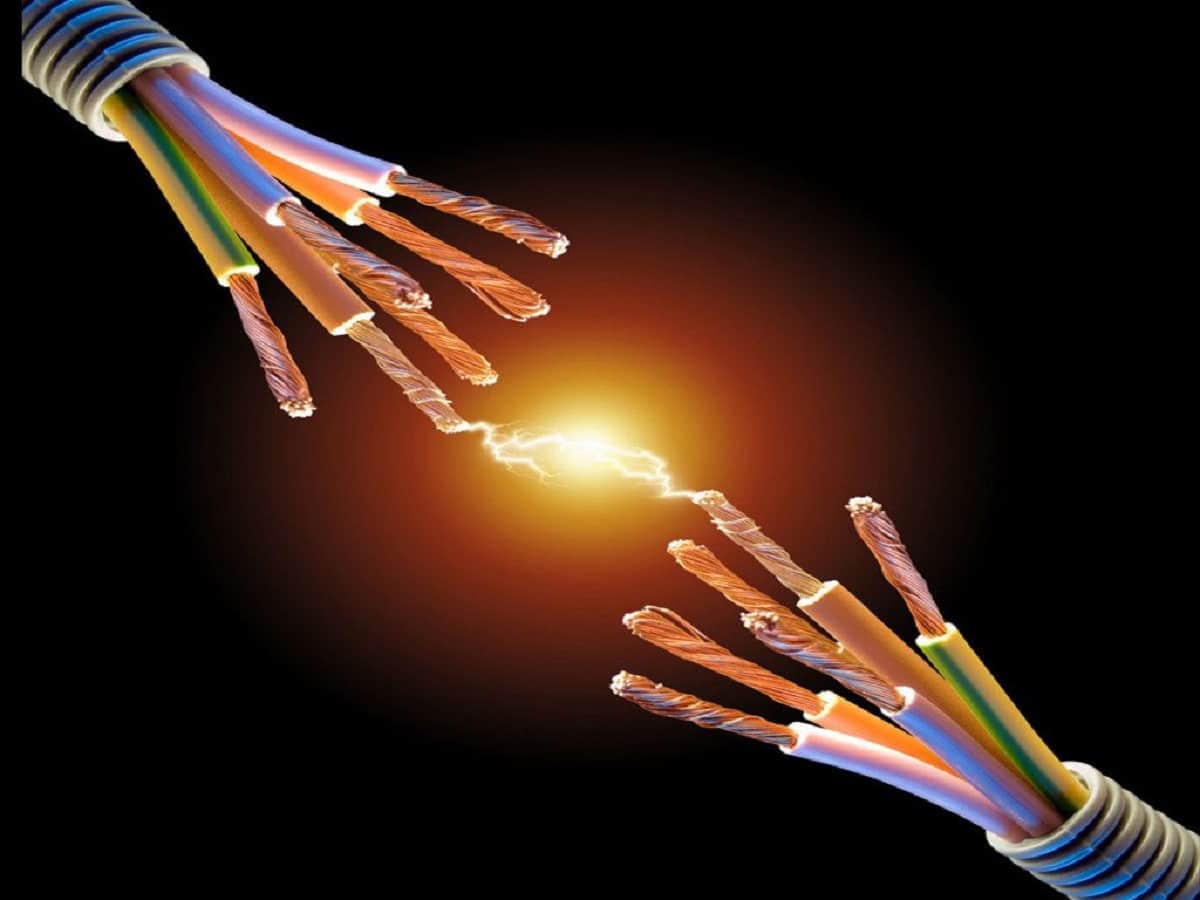
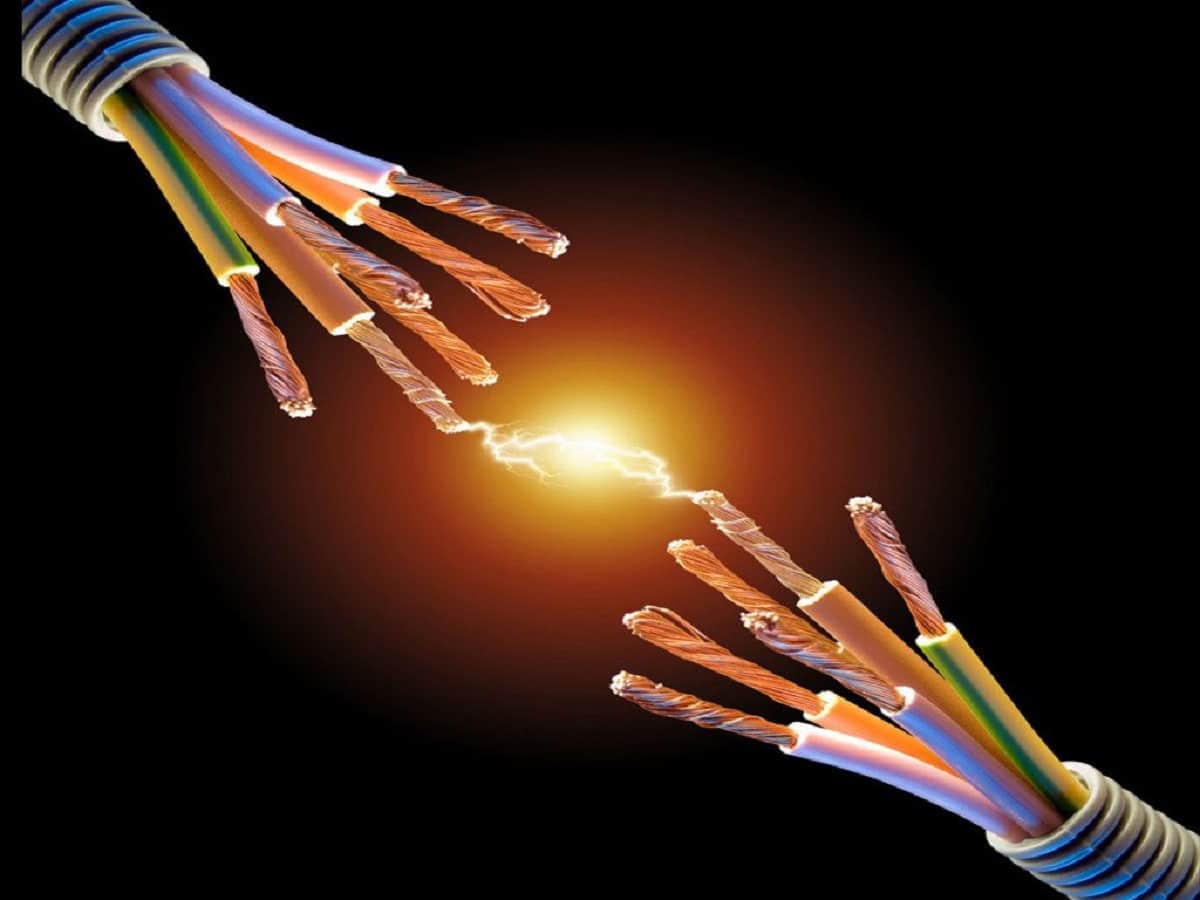
Science & Environment
Exploring The Relationship Between Temperature And Resistance In Conducting Materials
Modified: April 28, 2024
Explore the correlation between temperature and resistance in conducting materials. Learn about the impact on science and the environment. Discover more in this insightful study.
(Many of the links in this article redirect to a specific reviewed product. Your purchase of these products through affiliate links helps to generate commission for Temperatures.com, at no extra cost. Learn more)
Table of Contents
Introduction
The relationship between temperature and resistance in conducting materials is a fundamental concept in the field of physics and materials science. Understanding this relationship is crucial for various practical applications, ranging from electronic devices to industrial processes. As we delve into the intricate interplay between temperature and resistance, we uncover the profound impact of thermal energy on the behavior of conducting materials.
At the core of this relationship lies the concept of electrical resistance, which characterizes the opposition to the flow of electric current within a material. When temperature comes into play, it introduces a dynamic element that significantly influences the resistance of conducting materials. This phenomenon has far-reaching implications, shaping the design and functionality of numerous technological innovations.
Exploring the intricate dance between temperature and resistance unveils a captivating narrative of how materials respond to thermal stimuli. This journey takes us into the realm of quantum mechanics, where the vibrational motion of atoms and electrons within a material becomes a pivotal factor in determining its resistance at different temperatures. As we embark on this exploration, we unravel the intricate web of interactions that govern the behavior of conducting materials in the presence of varying thermal conditions.
The relationship between temperature and resistance in conducting materials serves as a gateway to a deeper understanding of the underlying physical principles that govern material properties. By delving into this relationship, we gain valuable insights into the behavior of materials under different thermal regimes, paving the way for innovative advancements in various fields.
As we embark on this exploration, we are poised to unravel the mysteries that lie at the intersection of temperature and resistance in conducting materials. This journey promises to illuminate the intricate mechanisms that underpin the behavior of materials, offering a glimpse into the fascinating world of thermal dynamics and material science.
Understanding Conducting Materials
Conducting materials, also known as conductors, are substances that allow the flow of electrical current with minimal resistance. This property is attributed to the presence of free electrons within the material, which are capable of moving in response to an applied electric field. The behavior of conducting materials is governed by the mobility of these free electrons, which enables the transmission of electrical energy through the material.
In the realm of solid-state physics, conducting materials are classified based on their electrical conductivity. Metals, such as copper and aluminum, are exemplary conductors due to their high electron mobility and abundance of free electrons. In contrast, semiconductors possess a lower conductivity compared to metals, as their electron mobility is more restricted. However, under specific conditions, semiconductors can exhibit conductive properties akin to those of metals.
The fundamental principle underlying the conductivity of materials lies in the movement of charge carriers, such as electrons, in response to an electric field. In conducting materials, the presence of free electrons allows for the transmission of electrical current with minimal hindrance. This characteristic sets them apart from insulating materials, which lack the abundance of free charge carriers necessary for effective conduction.
Furthermore, the atomic and molecular structure of conducting materials plays a crucial role in determining their electrical properties. The arrangement of atoms and the bonding characteristics within a material influence the mobility of free electrons, thereby impacting its overall conductivity. Additionally, the presence of impurities and defects within the material can affect its conductive behavior, leading to variations in electrical conductivity.
Understanding the behavior of conducting materials is essential for a wide array of applications, ranging from electrical wiring and circuitry to electronic components and power transmission. The ability of conducting materials to facilitate the flow of electrical current forms the backbone of modern technology, enabling the development of sophisticated electronic devices and power systems.
In essence, conducting materials represent a cornerstone of electrical engineering and materials science, serving as the building blocks for a myriad of technological innovations. By comprehending the intricate nature of these materials and their conductivity, scientists and engineers can harness their unique properties to drive advancements in diverse fields, from renewable energy technologies to telecommunications and beyond.
The Effect of Temperature on Resistance
The effect of temperature on resistance in conducting materials is a phenomenon deeply rooted in the fundamental principles of material science and quantum mechanics. At its core, this relationship stems from the intricate interplay between thermal energy and the behavior of electrons within a material. As temperature rises, the vibrational motion of atoms and electrons intensifies, leading to a notable impact on the material's resistance.
In metallic conductors, the relationship between temperature and resistance is characterized by an intriguing phenomenon known as the temperature coefficient of resistance. This coefficient quantifies the change in resistance per degree Celsius change in temperature and is a defining characteristic of conducting materials. Typically, for most metals, an increase in temperature leads to a corresponding increase in resistance. This behavior can be attributed to the heightened atomic vibrations induced by thermal energy, which impede the flow of electrons and, consequently, elevate the material's resistance.
In contrast, semiconducting materials exhibit a contrasting behavior with respect to temperature and resistance. As the temperature rises, the conductivity of semiconductors tends to increase, resulting in a decrease in resistance. This behavior is attributed to the release of additional charge carriers, such as electrons and holes, from their bound states, thereby enhancing the material's conductivity. The intricate dynamics of charge carrier mobility and thermal energy govern the temperature-resistance relationship in semiconductors, leading to their unique behavior in varying thermal conditions.
Furthermore, the effect of temperature on resistance extends beyond the macroscopic behavior of materials and delves into the realm of quantum mechanics. At the atomic and subatomic levels, the interaction between electrons and lattice vibrations, known as phonons, plays a pivotal role in shaping the material's resistance at different temperatures. This intricate interplay underscores the profound influence of quantum phenomena on the macroscopic behavior of conducting materials in the presence of thermal energy.
In essence, the effect of temperature on resistance in conducting materials embodies a captivating fusion of classical and quantum principles, offering a glimpse into the intricate mechanisms that govern material behavior. By unraveling this relationship, scientists and engineers gain valuable insights into the thermal dynamics of materials, paving the way for innovative advancements in diverse fields, from electronics and telecommunications to renewable energy and beyond.
Factors Affecting the Relationship Between Temperature and Resistance
The relationship between temperature and resistance in conducting materials is influenced by a myriad of factors that collectively shape the material's behavior in varying thermal conditions. These factors encompass both intrinsic properties of the material and external influences, offering a comprehensive understanding of the intricate interplay between temperature and resistance.
-
Material Composition: The elemental composition and crystalline structure of a conducting material profoundly impact its temperature-resistance relationship. Metals with distinct crystal lattices exhibit varying responses to temperature changes, with factors such as lattice defects and impurities influencing the material's resistance at different thermal regimes. Similarly, the band structure of semiconductors plays a pivotal role in determining their temperature-dependent conductivity, thereby affecting their resistance characteristics.
-
Electron Mobility: The mobility of free electrons within a conducting material is a critical factor in shaping its resistance-temperature relationship. As temperature increases, the vibrational motion of atoms and lattice ions intensifies, leading to enhanced scattering of free electrons. This scattering phenomenon impedes the flow of electrons, resulting in an increase in resistance for metallic conductors. Conversely, in semiconductors, the release of additional charge carriers at elevated temperatures can lead to a decrease in resistance, highlighting the intricate dynamics of electron mobility in response to thermal energy.
-
Band Gap Energy: In the case of semiconductors, the band gap energy, which represents the energy difference between the valence and conduction bands, significantly influences the temperature-resistance relationship. As temperature rises, the thermal energy can bridge the band gap, allowing electrons to transition from the valence band to the conduction band. This phenomenon leads to an increase in conductivity and a subsequent decrease in resistance, underscoring the profound impact of band gap energy on the material's behavior at different temperatures.
-
Temperature Coefficient of Resistance: The temperature coefficient of resistance, denoted by the symbol α, quantifies the rate of change in resistance with respect to temperature. This intrinsic property varies across different conducting materials and is a key determinant of their temperature-dependent resistance behavior. The temperature coefficient of resistance provides valuable insights into the material's response to thermal stimuli, serving as a crucial factor in understanding and predicting its resistance characteristics across a range of temperatures.
-
External Influences: External factors, such as applied voltage, current density, and environmental conditions, can also influence the relationship between temperature and resistance in conducting materials. The interplay between these external influences and the material's intrinsic properties adds a layer of complexity to the temperature-resistance relationship, highlighting the multifaceted nature of material behavior in real-world applications.
In essence, the relationship between temperature and resistance in conducting materials is governed by a complex interplay of intrinsic and extrinsic factors, offering a rich tapestry of phenomena that shape the material's behavior in response to thermal energy. By comprehensively understanding these factors, scientists and engineers can unravel the intricate dynamics of temperature-resistance relationships, paving the way for innovative advancements in diverse technological domains.
Applications of Temperature-Resistance Relationship in Conducting Materials
The temperature-resistance relationship in conducting materials underpins a myriad of practical applications across diverse technological domains. By leveraging the intrinsic behavior of materials in response to varying thermal conditions, engineers and scientists have unlocked innovative solutions that harness the dynamic interplay between temperature and resistance. These applications span a wide spectrum, ranging from electronic devices and sensors to industrial processes and renewable energy technologies.
Thermistors and Temperature Sensing
One of the prominent applications of the temperature-resistance relationship lies in the development of thermistors, which are temperature-sensitive resistors widely used for temperature sensing and control. Thermistors exhibit a significant change in resistance in response to temperature variations, making them invaluable for precise temperature measurement in electronic circuits, environmental monitoring systems, and thermal management applications. The nonlinear temperature-resistance characteristics of thermistors enable their utilization in temperature compensation circuits and thermal protection devices, contributing to the reliability and efficiency of electronic systems.
Heating Elements and Thermal Management
Conducting materials with tailored temperature-resistance characteristics find extensive use in heating elements and thermal management systems. The predictable response of conducting materials to temperature variations allows for the design of efficient heating elements for industrial processes, household appliances, and automotive applications. By modulating the resistance of conducting materials in response to temperature changes, engineers can achieve precise control over heating systems, contributing to energy efficiency and operational reliability. Additionally, the temperature-resistance relationship plays a crucial role in thermal management solutions, enabling the development of temperature-compensating devices and thermal control systems for diverse industrial and electronic applications.
Electronic Devices and Circuit Protection
In the realm of electronic devices and circuitry, the temperature-resistance relationship influences the design and performance of various components. Conducting materials with well-defined temperature-resistance characteristics are employed in circuit protection devices, such as thermal fuses and overcurrent protection elements, to safeguard electronic systems from temperature-induced damage and overloads. Furthermore, the temperature-dependent behavior of conducting materials is leveraged in the design of temperature-compensated circuits, ensuring the stability and reliability of electronic devices across a wide range of operating temperatures.
Renewable Energy Technologies
The temperature-resistance relationship in conducting materials plays a pivotal role in the advancement of renewable energy technologies, particularly in the realm of photovoltaics and energy conversion systems. Solar cells and photovoltaic modules utilize conducting materials with tailored temperature-resistance characteristics to optimize energy conversion efficiency and performance under varying environmental conditions. By understanding and manipulating the temperature-dependent behavior of conducting materials, researchers and engineers strive to enhance the reliability and longevity of renewable energy systems, contributing to the sustainable utilization of solar energy and other renewable resources.
Industrial Process Control and Sensing
In industrial automation and process control applications, the temperature-resistance relationship finds widespread use in sensing and control systems. Conducting materials with predictable temperature-resistance behavior are integrated into temperature sensors, thermal actuators, and control elements, enabling precise monitoring and regulation of industrial processes. The dynamic response of conducting materials to temperature variations facilitates the development of robust sensing and control solutions for diverse industrial applications, ranging from manufacturing and material processing to environmental monitoring and energy management.
In essence, the applications of the temperature-resistance relationship in conducting materials encompass a diverse array of technological domains, driving advancements in electronic systems, industrial processes, and renewable energy technologies. By harnessing the intrinsic behavior of materials in response to temperature changes, engineers and researchers continue to innovate and optimize solutions that leverage the dynamic interplay between temperature and resistance, shaping the landscape of modern technology and scientific exploration.
Conclusion
The intricate relationship between temperature and resistance in conducting materials embodies a captivating fusion of classical and quantum principles, offering a glimpse into the intricate mechanisms that govern material behavior. As we conclude this exploration, it becomes evident that the temperature-resistance relationship serves as a gateway to a deeper understanding of the underlying physical principles that govern material properties.
The effect of temperature on resistance in conducting materials is deeply rooted in the fundamental principles of material science and quantum mechanics. The interplay between thermal energy and the behavior of electrons within a material unveils a profound impact on the material's resistance. Whether in metallic conductors characterized by the temperature coefficient of resistance or in semiconductors exhibiting contrasting behavior, the influence of temperature on resistance reflects the intricate dynamics of charge carrier mobility and thermal energy.
Furthermore, the factors affecting the relationship between temperature and resistance, including material composition, electron mobility, band gap energy, temperature coefficient of resistance, and external influences, collectively shape the material's behavior in varying thermal conditions. This comprehensive understanding of intrinsic and extrinsic factors provides valuable insights into the intricate dynamics of temperature-resistance relationships, paving the way for innovative advancements in diverse technological domains.
The applications of the temperature-resistance relationship in conducting materials span a wide spectrum, ranging from electronic devices and sensors to industrial processes and renewable energy technologies. By leveraging the intrinsic behavior of materials in response to varying thermal conditions, engineers and scientists have unlocked innovative solutions that harness the dynamic interplay between temperature and resistance. These applications continue to drive advancements in electronic systems, industrial processes, and renewable energy technologies, shaping the landscape of modern technology and scientific exploration.
In essence, the exploration of the relationship between temperature and resistance in conducting materials offers a profound insight into the behavior of materials under different thermal regimes. By unraveling this relationship, scientists and engineers gain valuable insights into the thermal dynamics of materials, paving the way for innovative advancements in diverse fields, from electronics and telecommunications to renewable energy and beyond.