Home>Science & Environment>The Optimal Temperature Range For Enzyme Activity
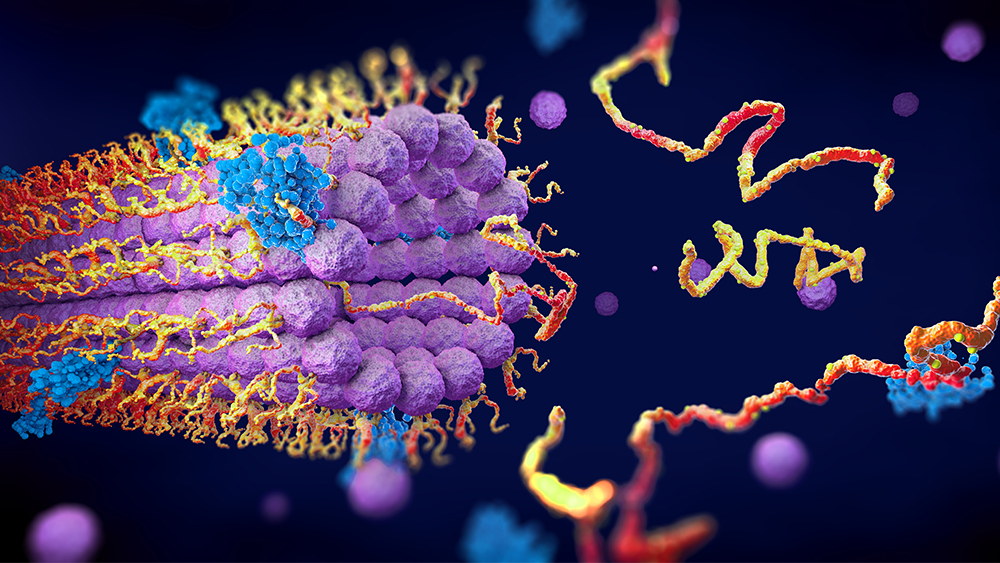
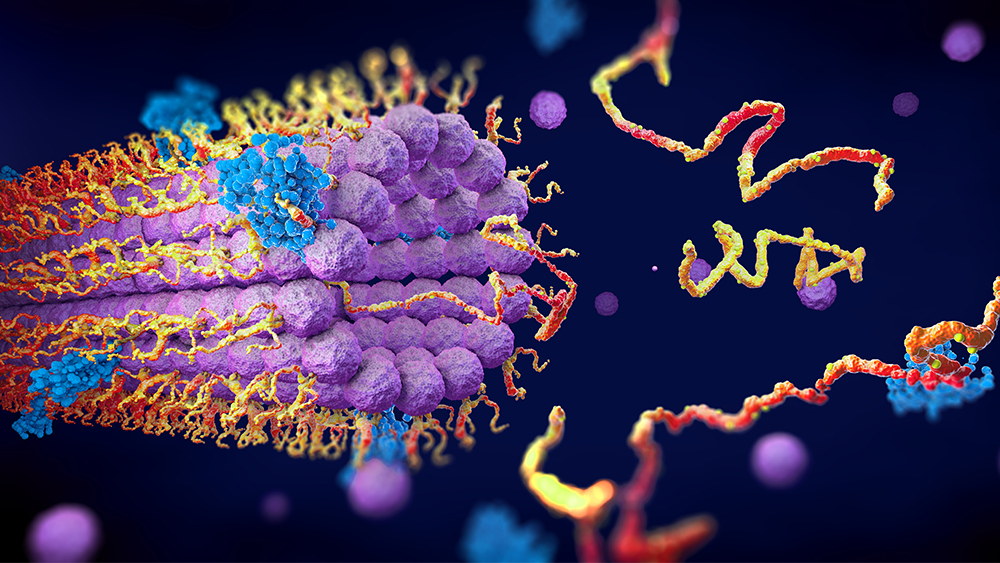
Science & Environment
The Optimal Temperature Range For Enzyme Activity
Published: March 3, 2024
Learn about the optimal temperature range for enzyme activity in this comprehensive guide. Explore the impact of temperature on biological processes. Ideal for science and environment enthusiasts.
(Many of the links in this article redirect to a specific reviewed product. Your purchase of these products through affiliate links helps to generate commission for Temperatures.com, at no extra cost. Learn more)
Table of Contents
Introduction
Enzymes are remarkable biological molecules that play a pivotal role in sustaining life. These catalysts facilitate and accelerate biochemical reactions within living organisms, enabling essential processes such as digestion, metabolism, and cellular respiration. Their significance extends across various fields, including medicine, food production, and environmental sustainability. Understanding the factors that influence enzyme activity, particularly temperature, is crucial for harnessing their potential in diverse applications.
Enzymes are highly specific in their function, with each type designed to catalyze a particular reaction. Their efficiency is profoundly influenced by environmental conditions, with temperature being a critical determinant. As temperature fluctuates, so does the rate of enzymatic reactions. This relationship between temperature and enzyme activity is a subject of extensive study and holds profound implications for numerous scientific disciplines.
In this article, we will delve into the intricate interplay between temperature and enzyme activity, exploring the optimal temperature range for maximal enzymatic function. By examining the effects of temperature on enzyme activity and the consequences of deviating from the optimal range, we aim to shed light on the delicate balance that governs enzymatic processes. This knowledge is fundamental for researchers, practitioners, and enthusiasts alike, as it underpins advancements in biotechnology, medicine, and ecological conservation.
As we embark on this exploration, it is essential to appreciate the intricate mechanisms that underlie enzymatic activity and the remarkable adaptability of enzymes to varying environmental conditions. By unraveling the complexities of temperature-dependent enzyme function, we can gain a deeper understanding of the delicate equilibrium that sustains life and fuels scientific innovation. Let us embark on this enlightening journey into the fascinating realm of enzyme activity and its intimate relationship with temperature.
Read more: Optimal Temperature Range For Succulents
Factors Affecting Enzyme Activity
Enzyme activity is intricately influenced by a myriad of factors, each exerting a distinct impact on the catalytic efficiency of these biological marvels. Understanding these factors is crucial for comprehending the dynamic nature of enzymatic processes and harnessing their potential in various applications. The key determinants of enzyme activity include temperature, pH, substrate concentration, enzyme concentration, and the presence of inhibitors or activators.
Temperature plays a pivotal role in modulating enzyme activity. As the temperature rises, the kinetic energy of molecules increases, leading to more frequent collisions between enzymes and substrates. This generally augments the rate of enzymatic reactions. However, excessively high temperatures can denature enzymes, disrupting their intricate three-dimensional structure and rendering them inactive. Conversely, lower temperatures reduce molecular motion, impeding the frequency of enzyme-substrate collisions and diminishing enzymatic activity.
pH, the measure of hydrogen ion concentration, profoundly influences enzyme activity. Each enzyme exhibits optimal activity at a specific pH range, dictated by its unique molecular structure and the nature of the catalyzed reaction. Deviations from this optimal pH can disrupt the ionic interactions and hydrogen bonding within the enzyme, leading to conformational changes that impair its functionality.
The concentration of substrates and enzymes significantly impacts enzymatic reactions. At low substrate concentrations, the rate of reaction is limited by the availability of substrates. As substrate concentration increases, the rate of reaction also rises until it reaches a point of saturation, where all enzyme molecules are engaged in catalysis. Similarly, varying enzyme concentrations directly influence the rate of reaction, with higher enzyme concentrations generally accelerating the reaction rate until saturation is achieved.
Inhibitors and activators are molecules that modulate enzyme activity. Inhibitors can bind to enzymes and impede their function, either reversibly or irreversibly. Conversely, activators enhance enzyme activity, often by binding to specific regulatory sites and inducing conformational changes that augment catalytic efficiency.
By comprehensively understanding these factors, researchers and practitioners can manipulate enzymatic processes to optimize their efficiency for diverse applications, ranging from industrial biocatalysis to therapeutic interventions. The intricate interplay of these factors underscores the remarkable adaptability and sensitivity of enzymes to their microenvironment, unveiling a captivating realm of biochemical intricacies.
Effects of Temperature on Enzyme Activity
Temperature exerts a profound influence on the catalytic efficiency of enzymes, dictating the rate of enzymatic reactions and ultimately shaping biological processes. As temperature rises, the kinetic energy of molecules escalates, leading to heightened molecular motion and increased collision frequency between enzymes and substrates. This generally augments the rate of enzymatic reactions, a phenomenon known as thermal activation. The enhanced kinetic energy facilitates the formation of enzyme-substrate complexes, accelerating the conversion of substrates into products.
However, the relationship between temperature and enzyme activity is intricate and delicate. While moderate temperature elevations enhance enzymatic reactions, excessively high temperatures can have detrimental effects. Enzymes are exquisitely sensitive to temperature fluctuations, and their three-dimensional structure is intricately linked to their functionality. Prolonged exposure to high temperatures can disrupt the intricate non-covalent interactions that maintain the enzyme's tertiary structure, leading to denaturation. This irreversible process alters the enzyme's conformation, rendering it inactive and incapable of catalyzing reactions.
Conversely, lower temperatures reduce molecular motion, impeding the frequency of enzyme-substrate collisions and diminishing enzymatic activity. At low temperatures, enzymes exhibit reduced flexibility and catalytic efficiency, as the molecular interactions necessary for substrate binding and catalysis are hindered by decreased kinetic energy.
The impact of temperature on enzyme activity is further compounded by the diversity of enzymes, each with its unique optimal temperature range. Some enzymes function optimally at moderate temperatures, while others thrive in extreme heat or cold. This diversity reflects the evolutionary adaptation of enzymes to the environmental conditions of their respective organisms, underscoring their remarkable versatility and resilience.
Understanding the effects of temperature on enzyme activity is pivotal for various scientific and practical endeavors. In biotechnological applications, such as industrial enzyme production and biocatalysis, optimizing temperature conditions is essential for maximizing enzymatic efficiency and productivity. Moreover, in the realm of medicine and healthcare, elucidating the temperature dependencies of enzymes is crucial for developing pharmaceutical interventions and diagnostic assays.
The intricate interplay between temperature and enzyme activity unveils the captivating intricacies of biochemical processes and underscores the pivotal role of environmental factors in shaping biological phenomena. By unraveling the effects of temperature on enzyme activity, researchers and practitioners can harness the potential of enzymes across diverse domains, driving innovation and advancements in science and technology.
Optimal Temperature Range for Enzyme Activity
The optimal temperature range for enzyme activity is a critical determinant of catalytic efficiency and biochemical processes. Each enzyme exhibits a specific temperature range within which it functions most effectively, reflecting its evolutionary adaptation to the environmental conditions of its host organism. This optimal range is intricately linked to the enzyme's structural stability, flexibility, and the kinetics of its catalytic reactions.
At the lower end of the temperature spectrum, enzymes exhibit reduced activity due to decreased molecular motion, which hinders the formation of enzyme-substrate complexes. As temperature rises within the optimal range, the kinetic energy of molecules escalates, leading to enhanced molecular motion and increased collision frequency between enzymes and substrates. This facilitates the catalytic conversion of substrates into products, maximizing enzymatic efficiency.
Conversely, as temperatures exceed the optimal range, the delicate balance of enzyme structure and function is compromised. High temperatures can induce thermal denaturation, disrupting the intricate non-covalent interactions that maintain the enzyme's three-dimensional structure. This results in the loss of enzymatic activity, as the altered conformation renders the enzyme incapable of catalyzing reactions effectively.
The optimal temperature range for enzyme activity is a manifestation of the enzyme's adaptation to the physiological conditions of its native environment. Enzymes derived from thermophilic organisms, which thrive in high-temperature environments, often exhibit optimal activity at elevated temperatures. In contrast, enzymes from psychrophilic organisms, adapted to cold habitats, function optimally at lower temperatures.
Understanding the optimal temperature range for enzyme activity is pivotal for numerous applications. In industrial biocatalysis, optimizing temperature conditions is essential for maximizing enzymatic efficiency and productivity. Moreover, in medical diagnostics and pharmaceutical development, knowledge of the temperature dependencies of enzymes is crucial for designing assays and formulating therapeutic interventions.
The optimal temperature range for enzyme activity encapsulates the delicate equilibrium that governs enzymatic processes, underscoring the remarkable adaptability and specificity of these biological catalysts. By elucidating the temperature dependencies of enzymes, researchers and practitioners can harness their potential across diverse domains, driving innovation and advancements in science and technology.
Consequences of Deviating from the Optimal Temperature Range
Deviation from the optimal temperature range for enzyme activity can have profound consequences, disrupting the delicate equilibrium that governs enzymatic processes and impeding essential biochemical reactions. When exposed to temperatures outside their optimal range, enzymes undergo structural alterations that significantly impact their functionality, leading to a cascade of biological repercussions.
Excessive heat can induce thermal denaturation, causing the disruption of the intricate non-covalent interactions that maintain the three-dimensional structure of enzymes. This structural perturbation renders the enzyme inactive, impeding its ability to catalyze reactions effectively. The consequences of denaturation extend beyond the immediate loss of enzymatic activity, as the altered conformation may render the enzyme susceptible to degradation or aggregation, further compromising its functionality.
Conversely, at lower temperatures, enzymes experience reduced molecular motion and flexibility, impeding the formation of enzyme-substrate complexes and diminishing catalytic efficiency. The sluggish enzymatic activity at suboptimal temperatures can hinder vital physiological processes, such as nutrient metabolism and cellular signaling, impacting the overall homeostasis of living organisms.
In practical applications, deviating from the optimal temperature range can have detrimental effects on industrial processes reliant on enzymatic catalysis. In biotechnological endeavors, such as the production of biofuels or the synthesis of pharmaceutical compounds, suboptimal temperature conditions can diminish enzymatic efficiency, leading to decreased productivity and increased production costs.
Moreover, in diagnostic assays and medical interventions, inaccuracies and inefficiencies may arise from temperature-induced deviations in enzyme activity. The reliability of diagnostic tests and the efficacy of therapeutic interventions are contingent on the precise functioning of enzymes, making it imperative to maintain optimal temperature conditions for enzymatic reactions.
The consequences of deviating from the optimal temperature range underscore the critical importance of temperature regulation in preserving enzymatic functionality and sustaining biological processes. By comprehending the repercussions of temperature-induced deviations, researchers and practitioners can implement strategies to mitigate these effects and optimize enzymatic performance across diverse applications.
The intricate interplay between temperature and enzyme activity unveils the captivating intricacies of biochemical processes and underscores the pivotal role of environmental factors in shaping biological phenomena. By unraveling the effects of temperature on enzyme activity, researchers and practitioners can harness the potential of enzymes across diverse domains, driving innovation and advancements in science and technology.
Read more: The Optimal Temperature Range For Cats
Conclusion
In conclusion, the intricate relationship between temperature and enzyme activity underscores the pivotal role of environmental factors in shaping biochemical processes. The effects of temperature on enzymatic reactions are profound and multifaceted, influencing the rate of catalysis and the structural integrity of enzymes. The optimal temperature range for enzyme activity represents a delicate equilibrium, reflecting the evolutionary adaptation of enzymes to the physiological conditions of their native environments.
Understanding the temperature dependencies of enzymes is paramount for diverse scientific and practical endeavors. In biotechnology, the optimization of temperature conditions is essential for maximizing enzymatic efficiency in industrial processes, ranging from biofuel production to pharmaceutical synthesis. Moreover, in the realm of healthcare and diagnostics, precise temperature regulation is crucial for ensuring the reliability and accuracy of enzymatic assays and therapeutic interventions.
The consequences of deviating from the optimal temperature range highlight the vulnerability of enzymes to temperature-induced perturbations and the far-reaching implications of such deviations. Thermal denaturation and reduced enzymatic activity at suboptimal temperatures can impede essential biochemical reactions, impacting industrial productivity, diagnostic accuracy, and therapeutic efficacy.
By unraveling the effects of temperature on enzyme activity, researchers and practitioners can harness the potential of enzymes across diverse domains, driving innovation and advancements in science and technology. The remarkable adaptability and specificity of enzymes to their microenvironment underscore their significance in sustaining life and fueling scientific progress.
In essence, the interplay between temperature and enzyme activity unveils the captivating intricacies of biochemical processes, emphasizing the critical role of environmental factors in shaping biological phenomena. By comprehending the temperature dependencies of enzymes, we can unlock the potential of these biological catalysts, paving the way for transformative developments in biotechnology, medicine, and ecological conservation.