Home>Science & Environment>Spontaneous Processes At Different Temperatures
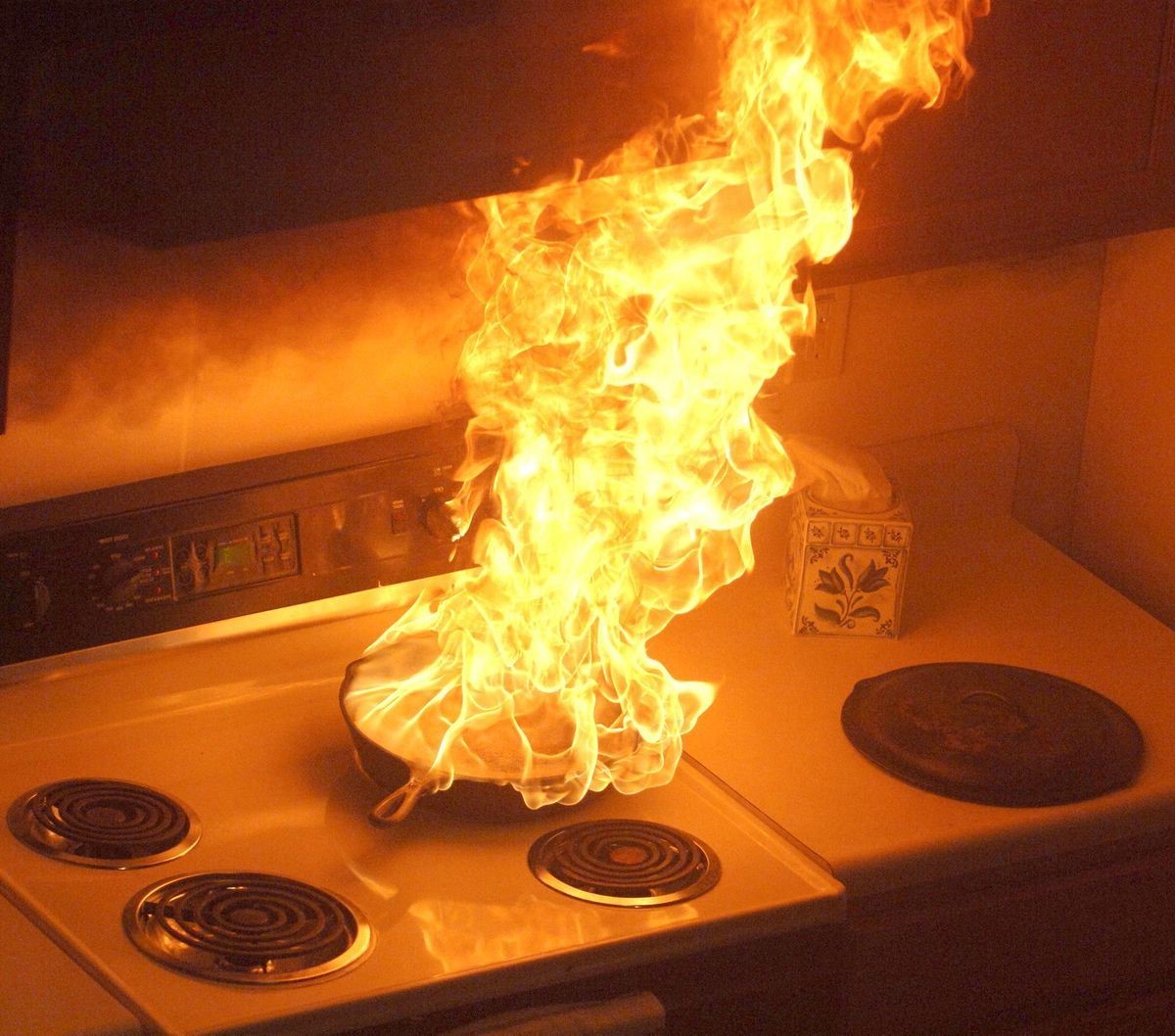
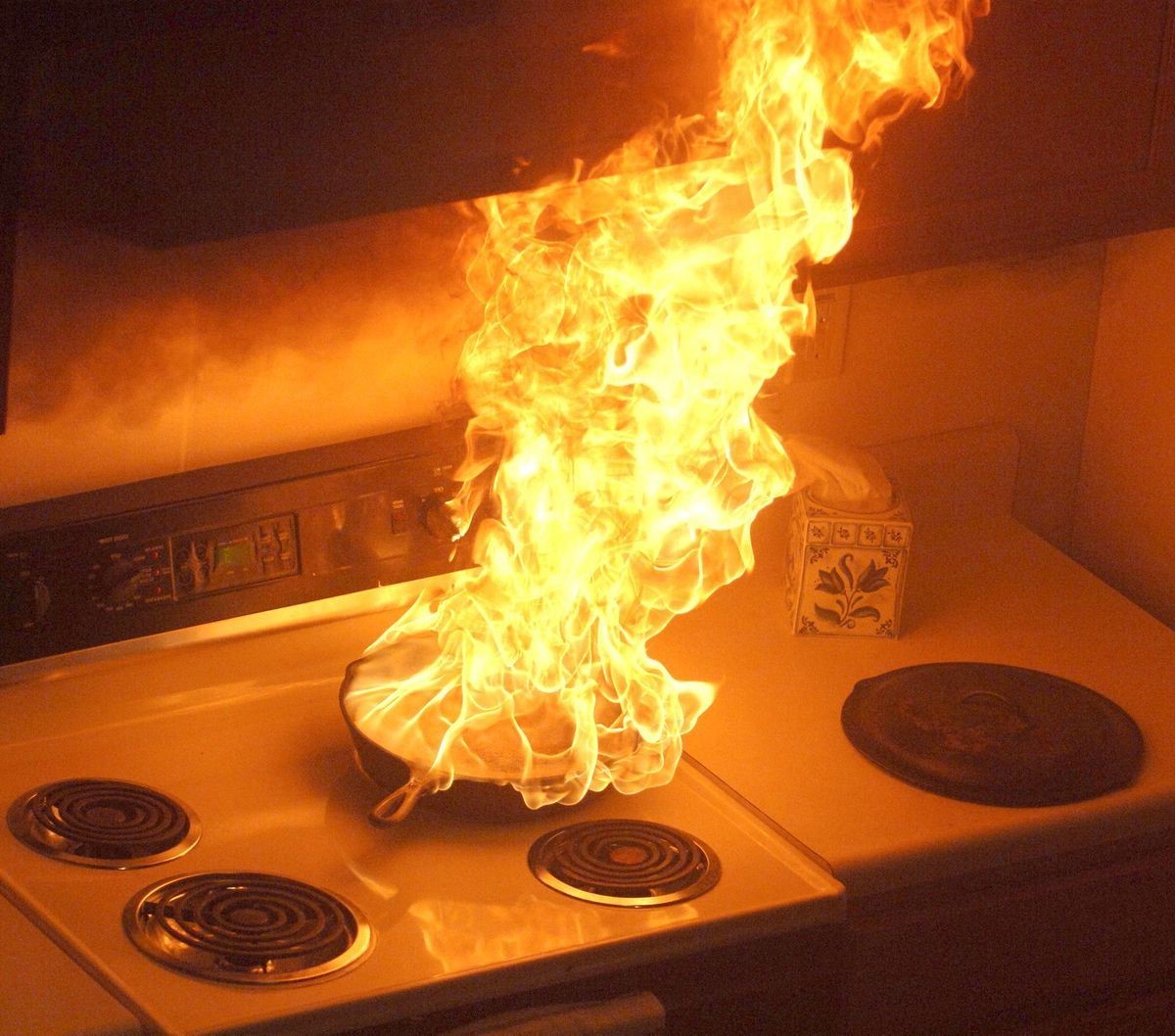
Science & Environment
Spontaneous Processes At Different Temperatures
Published: March 4, 2024
Explore the impact of temperature on spontaneous processes in the realm of science and the environment. Learn about the fascinating dynamics at play in various temperature conditions.
(Many of the links in this article redirect to a specific reviewed product. Your purchase of these products through affiliate links helps to generate commission for Temperatures.com, at no extra cost. Learn more)
Table of Contents
Understanding Spontaneous Processes
Spontaneous processes are natural, occurring without external intervention. They proceed on their own accord, driven by the inherent tendencies of the system. Understanding the factors that govern spontaneous processes is crucial in various scientific disciplines, particularly in the fields of thermodynamics and chemistry.
Spontaneous processes are characterized by an increase in entropy, a measure of the system's disorder or randomness. According to the second law of thermodynamics, the entropy of an isolated system tends to increase over time, leading to a greater degree of disorder. This concept is fundamental in understanding the directionality of natural processes.
In the context of chemical reactions, the spontaneity of a process is determined by the change in Gibbs free energy (∆G). If the ∆G value is negative, the process is spontaneous, indicating that the reaction can proceed without external energy input. Conversely, a positive ∆G value signifies a non-spontaneous process, requiring an external driving force to occur.
Moreover, the spontaneity of a process is closely linked to the enthalpy (∆H) and entropy (∆S) changes. While a decrease in enthalpy favors spontaneity, an increase in entropy strongly drives spontaneous processes. This interplay between enthalpy and entropy highlights the intricate balance of energy and disorder in determining the natural progression of physical and chemical transformations.
Understanding spontaneous processes provides valuable insights into the behavior of various systems, from the dissolution of solutes in solvents to the combustion of fuels. By delving into the underlying principles governing spontaneity, scientists and researchers can unravel the intricacies of natural phenomena, paving the way for innovative advancements and a deeper comprehension of the world around us.
Temperature and Entropy Change
Temperature plays a pivotal role in influencing the entropy change of a system. As the temperature increases, the entropy of a substance also tends to rise. This relationship is elucidated by the Boltzmann equation, which states that entropy is directly proportional to the natural logarithm of the number of accessible microstates for a given system. In essence, an increase in temperature leads to a greater distribution of energy among the particles, resulting in a higher number of possible arrangements and, consequently, an elevation in entropy.
The connection between temperature and entropy change is particularly evident in phase transitions. When a substance undergoes a phase change, such as from a solid to a liquid or from a liquid to a gas, the entropy of the system experiences a substantial increase. This phenomenon is attributed to the greater freedom of movement and arrangement of particles at higher temperatures, leading to a surge in disorder and randomness within the system.
Furthermore, the relationship between temperature and entropy change is integral to the understanding of the spontaneity of physical and chemical processes. In the context of thermodynamics, the concept of absolute temperature, denoted by the symbol T, is directly linked to the entropy change (∆S) through the equation ∆S = q_rev/T, where q_rev represents the reversible heat transfer. This equation underscores the fundamental role of temperature in governing the direction and magnitude of entropy changes.
At the molecular level, the influence of temperature on entropy change is intricately tied to the vibrational, rotational, and translational motions of particles. As the temperature increases, the kinetic energy of the particles escalates, leading to heightened molecular motion and a greater number of accessible energy states. Consequently, the system's entropy surges, reflecting the augmented disorder and randomness associated with the heightened thermal agitation of particles.
In summary, the relationship between temperature and entropy change is a fundamental aspect of thermodynamics, elucidating the profound impact of temperature variations on the disorder and randomness of systems. By comprehending this intricate interplay, scientists and researchers can gain deeper insights into the behavior of diverse substances and processes, ultimately advancing our understanding of the natural world and its underlying principles.
Effect of Temperature on Gibbs Free Energy
The effect of temperature on Gibbs free energy is a critical aspect of thermodynamics, delineating the profound influence of thermal energy on the spontaneity of physical and chemical processes. Gibbs free energy, denoted by the symbol G, represents the maximum amount of non-expansion work that can be extracted from a closed system at constant temperature and pressure. It serves as a pivotal indicator of the feasibility and directionality of a given process, offering valuable insights into the energetic favorability of spontaneous transformations.
Temperature exerts a significant impact on Gibbs free energy through its influence on the enthalpy (∆H) and entropy (∆S) changes. The relationship is encapsulated in the equation ∆G = ∆H – T∆S, where T represents the absolute temperature. As temperature varies, the interplay between enthalpy and entropy changes becomes increasingly pronounced, leading to distinct alterations in the Gibbs free energy of the system.
At higher temperatures, the contribution of entropy to the overall Gibbs free energy becomes more substantial. This is attributed to the heightened thermal agitation of particles, which amplifies the disorder and randomness within the system. As a result, the T∆S term in the Gibbs free energy equation becomes more pronounced, exerting a greater influence on the overall ∆G value. Consequently, processes characterized by a positive ∆S value are more likely to exhibit a negative ∆G at elevated temperatures, indicating a heightened propensity for spontaneity.
Conversely, at lower temperatures, the enthalpic contribution to Gibbs free energy assumes greater significance. The decrease in thermal energy leads to a diminished impact of entropy on the overall ∆G value. Consequently, processes with a negative ∆H value are more likely to display a negative ∆G at lower temperatures, underscoring the pivotal role of enthalpy in driving spontaneity under such conditions.
The effect of temperature on Gibbs free energy underscores the intricate balance between enthalpy and entropy changes in governing the spontaneity of diverse processes. By comprehending the nuanced interplay between temperature and Gibbs free energy, scientists and researchers can gain deeper insights into the energetic underpinnings of natural phenomena, paving the way for innovative advancements in various scientific disciplines.
Spontaneous Processes at Low Temperatures
At low temperatures, the interplay between thermodynamic parameters exerts a profound influence on the spontaneity of physical and chemical processes. The impact of temperature on entropy change and Gibbs free energy becomes particularly pronounced under such conditions, shaping the feasibility and directionality of diverse transformations.
When the temperature decreases, the thermal energy of the system diminishes, leading to a reduction in the kinetic energy of particles. Consequently, the molecular motion and the number of accessible energy states decrease, resulting in a decline in entropy. This reduction in entropy at low temperatures underscores the diminished disorder and randomness within the system, exerting a pivotal influence on the spontaneity of processes.
In the context of Gibbs free energy, the effect of temperature at lower temperatures is characterized by the heightened significance of enthalpy in governing the spontaneity of transformations. With the diminished thermal agitation of particles, the contribution of entropy to the overall Gibbs free energy becomes less pronounced. As a result, processes characterized by a negative ∆H value are more likely to exhibit a negative ∆G at lower temperatures, indicating a heightened propensity for spontaneity driven by enthalpic favorability.
Furthermore, at low temperatures, the reduction in thermal energy leads to a diminished kinetic barrier for certain reactions, particularly those involving the formation of ordered structures. This phenomenon is exemplified in the crystallization of substances, where the decreased thermal motion facilitates the organization of particles into a more ordered state. Consequently, processes such as crystallization exhibit a heightened propensity for spontaneity at lower temperatures, driven by the enthalpic favorability and the reduced thermal disorder within the system.
In summary, at low temperatures, the diminished thermal energy engenders distinct alterations in the spontaneity of processes, accentuating the pivotal role of enthalpy in driving favorable transformations. By comprehending the intricate interplay between temperature, entropy change, and Gibbs free energy at low temperatures, scientists and researchers can gain deeper insights into the thermodynamic underpinnings of diverse phenomena, paving the way for innovative advancements in the realm of physical and chemical transformations.
Spontaneous Processes at High Temperatures
At high temperatures, the behavior of physical and chemical systems undergoes profound transformations, driven by the interplay between thermodynamic parameters. The influence of temperature on entropy change and Gibbs free energy assumes heightened significance under such conditions, shaping the spontaneity and directionality of diverse processes.
As the temperature escalates, the thermal energy of the system surges, leading to a heightened kinetic energy of particles. This augmented thermal agitation engenders a greater degree of molecular motion and an increased number of accessible energy states, culminating in a surge in entropy. The heightened disorder and randomness within the system at high temperatures underscore the pivotal role of entropy in driving spontaneous processes, reflecting the profound impact of thermal energy on the feasibility of diverse transformations.
In the context of Gibbs free energy, the effect of temperature at high temperatures is characterized by the heightened significance of entropy in governing the spontaneity of processes. With the amplified thermal motion of particles, the contribution of entropy to the overall Gibbs free energy becomes more pronounced. Consequently, processes characterized by a positive ∆S value are more likely to exhibit a negative ∆G at elevated temperatures, indicating a heightened propensity for spontaneity driven by the entropic favorability.
Moreover, at high temperatures, certain reactions experience a diminished kinetic barrier, particularly those involving the disruption of ordered structures. This phenomenon is exemplified in the melting of solids, where the heightened thermal energy leads to the breakdown of ordered arrangements, facilitating the transition to a more disordered state. Consequently, processes such as melting exhibit a heightened propensity for spontaneity at high temperatures, driven by the entropic favorability and the amplified thermal disorder within the system.
In summary, at high temperatures, the heightened thermal energy engenders distinct alterations in the spontaneity of processes, accentuating the pivotal role of entropy in driving favorable transformations. By comprehending the intricate interplay between temperature, entropy change, and Gibbs free energy at high temperatures, scientists and researchers can gain deeper insights into the thermodynamic underpinnings of diverse phenomena, paving the way for innovative advancements in the realm of physical and chemical transformations.